Introduction
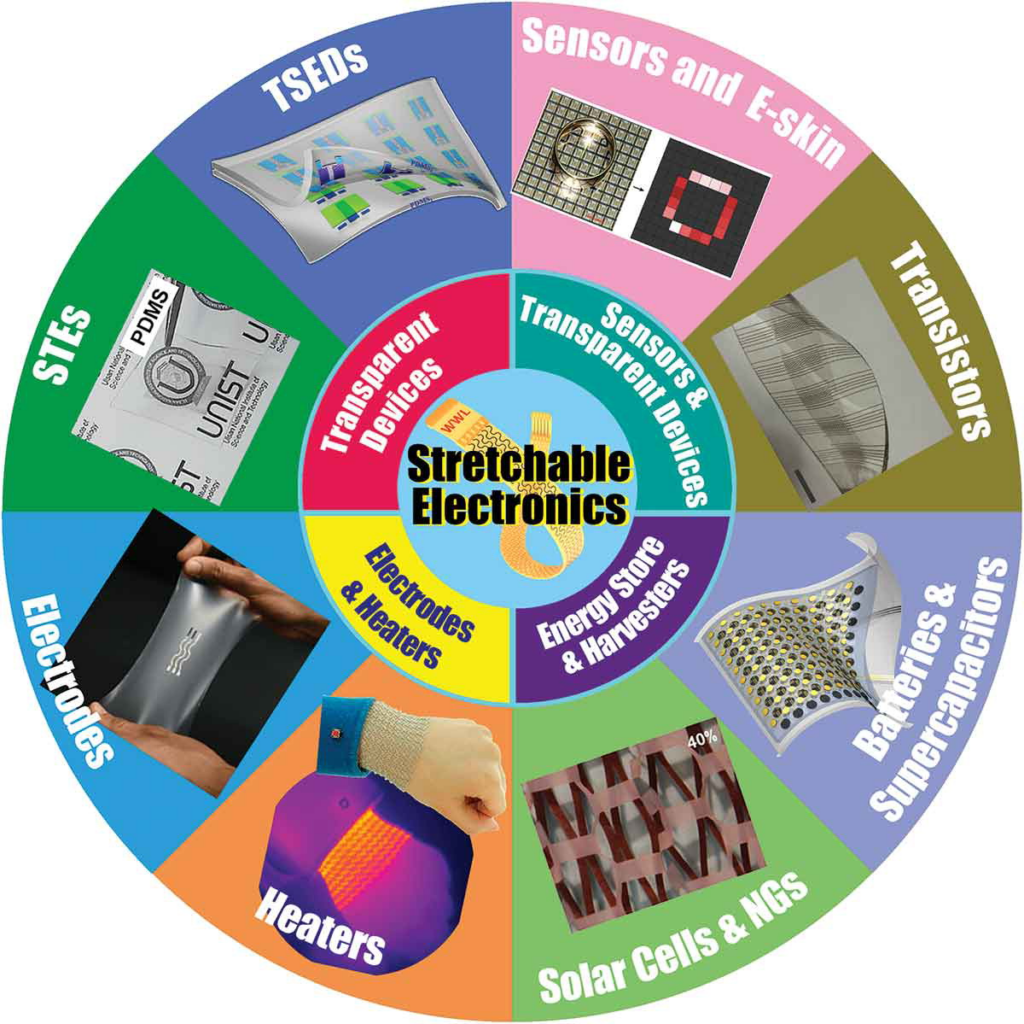
The rapid advancement of electronics has paved the way for more integrated and versatile systems in everyday life. Among the most exciting developments are flexible and stretchable electronics, which represent a revolutionary step forward in creating devices that can bend, stretch, and conform to various surfaces. These new forms of electronics have the potential to transform industries such as wearable technology, healthcare, automotive design, and defense, where traditional rigid electronics often fall short.
Flexible electronics are reshaping how we interact with technology, enabling more comfortable, efficient, and adaptive systems. Stretchable electronics, in particular, push the boundaries of design, allowing devices to adapt to the human body, clothing, or even biological tissues. This article explores how flexible and stretchable electronics work, the technology behind them, their uses, and the advantages and disadvantages they offer.
How Flexible, Stretchable Electronics Work
Flexible and stretchable electronics rely on a combination of innovative materials and unique design architectures that allow electronic components to function while being bent, twisted, or stretched. The core concept behind these devices is to combine conductive materials with substrates that can deform without compromising electrical performance. Here’s a breakdown of how these technologies work:
- Flexible Substrates:
- Flexible electronics are typically built on thin, bendable substrates made from materials like polymer films, paper, or metal foils. These materials are capable of bending and conforming to various shapes without breaking. Common examples include polyimide, PET (polyethylene terephthalate), or even graphene, which can provide flexibility while retaining excellent electrical conductivity.
- Stretchable Conductive Materials:
- The conductive materials used in stretchable electronics need to maintain performance even when stretched. Silver nanowires, carbon nanotubes (CNTs), conductive polymers, and liquid metals (like gallium or indium-gallium alloys) are often used in the construction of stretchable circuits. These materials have properties that allow them to deform without breaking or losing conductivity, making them ideal for applications where flexibility is essential.
- Design Architecture:
- Stretchable electronics use specialized circuit designs that distribute mechanical strain over a large area, such as the zigzag or serpentine patterns used for wiring. These patterns enable the materials to stretch and flex without causing the circuit to break. When the material is deformed, the strain is spread out, preventing the breaking of conductive pathways.
- Integration with Other Technologies:
- Flexible and stretchable electronics can be integrated with a variety of other technologies, including sensors, OLED (organic light-emitting diode) displays, solar cells, and even batteries. They can be combined with sensors for health monitoring, or used as flexible displays on wearable devices like smartwatches or fitness trackers.
Technology Behind Flexible, Stretchable Electronics
Several technologies and innovations are at the core of developing flexible and stretchable electronics. These include:
- Organic Electronics:
- Many flexible devices use organic semiconductors, which are carbon-based compounds capable of conducting electricity. Organic materials like organic light-emitting diodes (OLEDs) and organic photovoltaics (OPVs) are crucial for flexible, stretchable electronics due to their lightweight, bendable nature and relatively simple fabrication processes.
- Nanomaterials:
- Carbon nanotubes (CNTs) and graphene are pivotal in achieving the required conductivity and mechanical properties for stretchable electronics. These materials can be processed into flexible films that maintain their electrical properties even when subjected to mechanical deformation.
- Flexible Sensors:
- Flexible and stretchable sensors made from conductive polymers or nanomaterials are integrated into electronic systems to measure pressure, temperature, motion, and biological signals (such as heart rate or brain activity). These sensors are essential for creating the next generation of wearables and health-monitoring systems.
- Printing and Fabrication Techniques:
- 3D printing and roll-to-roll printing technologies allow the mass production of flexible electronics, enabling scalable, low-cost manufacturing. These techniques are used to print electronic circuits, sensors, and even solar cells onto flexible substrates, allowing for high-volume production of stretchable, flexible systems.
- Hybrid Materials:
- Hybrid materials are often used to combine the benefits of both rigid and flexible electronics. For example, incorporating flexible substrates into traditionally rigid materials, such as metals and ceramics, can create devices that combine the best properties of both worlds.
Uses of Flexible, Stretchable Electronics
Flexible and stretchable electronics open the door to a wide range of applications that were previously not possible with traditional rigid electronics. Some of the primary uses include:
- Wearable Technology:
- Flexible electronics are perfect for wearable devices like smartwatches, fitness trackers, smart clothing, and health-monitoring patches. These devices can conform to the body’s shape and movement, providing real-time data on health parameters like heart rate, blood pressure, or oxygen levels without sacrificing comfort.
- Medical Devices:
- Stretchable electronics are used in the development of biomedical devices that can adhere to the skin or even integrate with the human body. For example, stretchable sensors can be worn on the skin to monitor vital signs or biosensors that detect disease biomarkers. Moreover, flexible bioelectronics can be used in prosthetics, offering enhanced adaptability for individuals with disabilities.
- Flexible Displays:
- OLED screens are one of the most well-known examples of flexible electronics used in display technology. Flexible displays are used in smartphones, tablets, TVs, and even foldable or rollable screens, where the display can be bent, folded, or rolled up without breaking.
- Energy Harvesting:
- Flexible, stretchable electronics are also used in energy harvesting systems that convert movement, heat, or sunlight into electrical energy. Flexible solar cells and piezoelectric devices can be integrated into wearable technology or everyday objects, offering continuous energy collection without the need for rigid solar panels or power sources.
- Defense and Military Applications:
- In the military, flexible and stretchable electronics are being developed for uniforms that monitor soldiers’ health, integrate communications systems, or provide real-time battlefield data. These electronics are designed to be lightweight, durable, and adaptable to extreme environments.
Advantages of Flexible, Stretchable Electronics
- Lightweight and Comfortable:
- Flexible electronics can be made to be lightweight, making them ideal for wearable devices that need to be comfortable for long-term use. They are particularly beneficial for devices worn on the body, as they do not add significant bulk or weight.
- Durable and Resilient:
- Stretchable electronics are highly resilient and can withstand bending, stretching, and folding without breaking or losing functionality. This makes them ideal for applications that require durability in challenging environments, such as wearable health monitors or outdoor devices.
- Versatility in Design:
- Flexible electronics offer greater design freedom, allowing for the creation of devices that conform to the body or other curved surfaces. This opens up possibilities for customized products, like smart fabrics that can integrate sensors, displays, and even heating/cooling elements into clothing.
- Reduced Production Costs:
- With technologies like 3D printing and roll-to-roll printing, flexible electronics can be produced at a lower cost than traditional rigid electronics. This could lead to more affordable wearable devices and other electronics for consumers and industries alike.
- Integration with New Technologies:
- Flexible and stretchable electronics can be integrated with other emerging technologies such as artificial intelligence (AI), machine learning, and augmented reality (AR). This opens up new possibilities for creating smart, adaptive systems for healthcare, education, and entertainment.
Disadvantages of Flexible, Stretchable Electronics
- Limited Lifetime:
- Despite their durability, flexible and stretchable electronics may have a shorter lifespan compared to traditional rigid electronics. Over time, materials may degrade with constant stretching or bending, leading to a loss of functionality or performance.
- Performance Limitations:
- Flexible electronics can sometimes suffer from lower performance than their rigid counterparts, especially in terms of power density or processing speed. While they are suitable for low-power applications, more demanding uses (such as high-speed computing) may still require traditional rigid materials.
- Manufacturing Complexity:
- While production costs are expected to be lower in the future, the manufacturing processes for flexible and stretchable electronics are still more complex and expensive compared to traditional electronics. High-volume production still faces hurdles related to material consistency, durability, and reliability.
- Sensitivity to Environmental Factors:
- Flexible electronics can be more sensitive to temperature changes, humidity, and other environmental factors. This could affect their performance, especially in outdoor or harsh conditions, where traditional electronics tend to be more stable.
- Material Limitations:
- The materials used in flexible electronics, such as conductive polymers or nanomaterials, may still face issues like scalability, cost, and long-term stability. Further research and development are needed to optimize these materials for broad use in consumer devices.
Conclusion
Flexible and stretchable electronics are setting the stage for the next wave of wearable devices, smart clothing, health-monitoring systems, and more. With their ability to bend, stretch, and conform to various surfaces, these advanced materials promise to enhance user experience, performance, and comfort across a wide range of applications. While there are challenges, including durability and performance limitations, the future of flexible electronics looks promising, especially as material science and manufacturing processes continue to evolve. As the technology matures, we can expect to see these systems become an integral part of daily life, offering new levels of flexibility, functionality, and innovation.