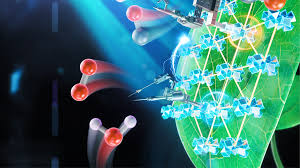
Introduction:
Artificial photosynthesis is an emerging field of research that seeks to replicate the natural process of photosynthesis, where plants convert sunlight, water, and carbon dioxide into oxygen and glucose. The goal of artificial photosynthesis is to create a sustainable method for producing clean energy, such as hydrogen or other fuels, while also helping to reduce the levels of carbon dioxide in the atmosphere. By mimicking the way plants capture solar energy, scientists hope to develop a renewable energy source that can mitigate climate change, reduce dependency on fossil fuels, and support the transition to a low-carbon economy.
This process holds the potential to revolutionize energy production and create a cleaner, more sustainable future.
How It Works:
Artificial photosynthesis works by using sunlight to drive chemical reactions that produce energy, much like the natural process occurring in plants. However, the artificial version is typically designed to produce usable fuels, such as hydrogen or hydrocarbons, rather than just oxygen and glucose. The process can be broken down into a few basic steps:
- Light Absorption: The system uses light-absorbing materials, usually semiconductors or photocatalysts, to capture sunlight. These materials convert the light energy into electronic energy (i.e., electricity).
- Water Splitting: Similar to natural photosynthesis, artificial photosynthesis typically splits water (H2O) into oxygen (O2) and hydrogen (H2). The hydrogen can then be stored and used as a clean fuel, while the oxygen is released as a byproduct.
- Carbon Dioxide Reduction: In some artificial photosynthesis systems, carbon dioxide (CO2) is also captured from the atmosphere and converted into organic compounds, such as methane or other hydrocarbons, using the energy from sunlight. This process is akin to how plants convert CO2 into glucose.
- Energy Storage: The hydrogen or other fuels produced through artificial photosynthesis can be stored and used later in fuel cells, vehicles, or power plants, providing a clean, renewable energy source.
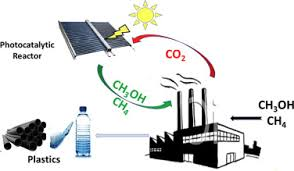
Technology Used:
Several advanced technologies are being used to achieve artificial photosynthesis, each aiming to efficiently replicate the natural process of photosynthesis:
- Photocatalysts: These are materials that facilitate chemical reactions under the influence of light. Semiconductors, such as titanium dioxide (TiO2), are commonly used as photocatalysts in artificial photosynthesis. These materials can absorb sunlight and trigger reactions that split water molecules into hydrogen and oxygen.
- Photoelectrochemical Cells (PECs): PECs are devices that combine light-absorbing materials with electrodes to convert solar energy into chemical energy. In artificial photosynthesis, PECs are used to split water molecules or reduce CO2. They essentially act as artificial “leaves” that directly convert sunlight into storable fuels.
- Solar Fuels: One of the most promising technologies in artificial photosynthesis is the development of solar fuels. These fuels, like hydrogen or synthetic hydrocarbons, are produced directly from sunlight through chemical processes such as water splitting or CO2 reduction. These fuels can be used in a variety of applications, including transportation and power generation.
- Biomimicry: Many artificial photosynthesis systems are designed using biomimicry, meaning they mimic the structures and processes found in nature. For example, researchers have developed biohybrid systems that integrate biological components, such as enzymes, with synthetic materials to enhance efficiency and mimic the natural process of photosynthesis.
- Electrolyzers and Fuel Cells: Electrolyzers are used to separate water into hydrogen and oxygen, which is the key step in producing hydrogen fuel. Once produced, the hydrogen can be stored and later used in fuel cells, which convert hydrogen into electricity in a clean and efficient manner.
Advantages:
Artificial photosynthesis offers several significant advantages, especially in the context of environmental sustainability and clean energy:
- Clean Energy Production: One of the main benefits of artificial photosynthesis is its potential to produce clean, renewable energy. By mimicking the natural process of photosynthesis, it offers a carbon-neutral method of generating hydrogen and other fuels.
- Reduction of Carbon Emissions: Through the process of carbon dioxide reduction, artificial photosynthesis could help reduce atmospheric CO2 levels, thereby mitigating climate change. By converting CO2 into useful fuels, it addresses two global issues simultaneously—energy production and carbon emissions.
- Sustainability: The technology relies on abundant resources—sunlight, water, and carbon dioxide—making it highly sustainable and eco-friendly. It has the potential to generate energy in a manner that doesn’t deplete natural resources or contribute to environmental degradation.
- Energy Storage: Artificial photosynthesis can produce fuels like hydrogen that can be stored and transported, unlike solar power, which must be used immediately or stored in batteries. This offers the potential for large-scale energy storage and a continuous supply of power, even when the sun isn’t shining.
- Energy Independence: The widespread adoption of artificial photosynthesis could reduce reliance on fossil fuels and imported energy sources, providing a pathway to energy independence for nations and regions.
Disadvantages:
While artificial photosynthesis holds great promise, several challenges must be addressed before it can be widely implemented:
- Efficiency: Currently, artificial photosynthesis systems are not as efficient as natural photosynthesis in terms of energy conversion. The conversion of sunlight into usable energy through artificial systems is still relatively low, and significant advancements are needed to improve efficiency.
- High Costs: The technology behind artificial photosynthesis, including photocatalysts, electrochemical cells, and biohybrid systems, can be expensive to develop and manufacture. This makes it less commercially viable at present compared to other renewable energy technologies, such as solar or wind power.
- Scalability: Scaling up artificial photosynthesis to a level that could meet global energy demands is another major hurdle. The current technology is primarily at the laboratory or small-scale prototype stage, and scaling it to industrial levels will require substantial advancements in materials, engineering, and infrastructure.
- Material Challenges: Many of the materials used in artificial photosynthesis, such as semiconductors and photocatalysts, are still not optimal in terms of durability, cost, and performance. Developing materials that are both highly efficient and cost-effective is a key challenge.
- Storage and Distribution: While hydrogen is a promising fuel, its storage and transportation present logistical challenges. Hydrogen requires specialized infrastructure, such as high-pressure tanks or pipelines, and the energy required to store and transport hydrogen can reduce the overall efficiency of the system.
Future Scope:
The future of artificial photosynthesis holds great potential, especially in the context of energy production, climate change mitigation, and sustainable development:
- Technological Advancements: Continued research and development will likely lead to more efficient systems. Scientists are exploring new photocatalysts, more effective photoelectrochemical cells, and alternative materials that could enhance the performance and cost-effectiveness of artificial photosynthesis.
- Commercial Viability: As technology advances and costs come down, artificial photosynthesis could become a commercially viable option for large-scale energy production. This could help meet growing energy demands without the negative environmental impacts of fossil fuels.
- Integration with Other Renewable Sources: Artificial photosynthesis could be integrated into existing renewable energy systems, such as solar and wind power, to create a more reliable and balanced energy grid. Hydrogen produced via artificial photosynthesis could be stored and used in fuel cells to supplement other renewable energy sources.
- Carbon Capture: The dual benefit of producing clean energy while reducing atmospheric CO2 through carbon capture could make artificial photosynthesis a crucial technology in combating climate change. This could help countries meet their carbon reduction goals under international climate agreements.
- Global Adoption: As artificial photosynthesis becomes more efficient and scalable, it could be deployed worldwide, providing a sustainable and decentralized solution to energy production. It could help reduce global dependence on fossil fuels and provide clean, renewable energy to underserved regions.